General principles of solar ethanol production
- Dmitry Vinograd
- Oct 30, 2023
- 15 min read
Updated: Oct 30, 2023
Memorandum:
General principles of solar ethanol production and plant design and related research activities based on experience in Joule Unlimited Technologies.
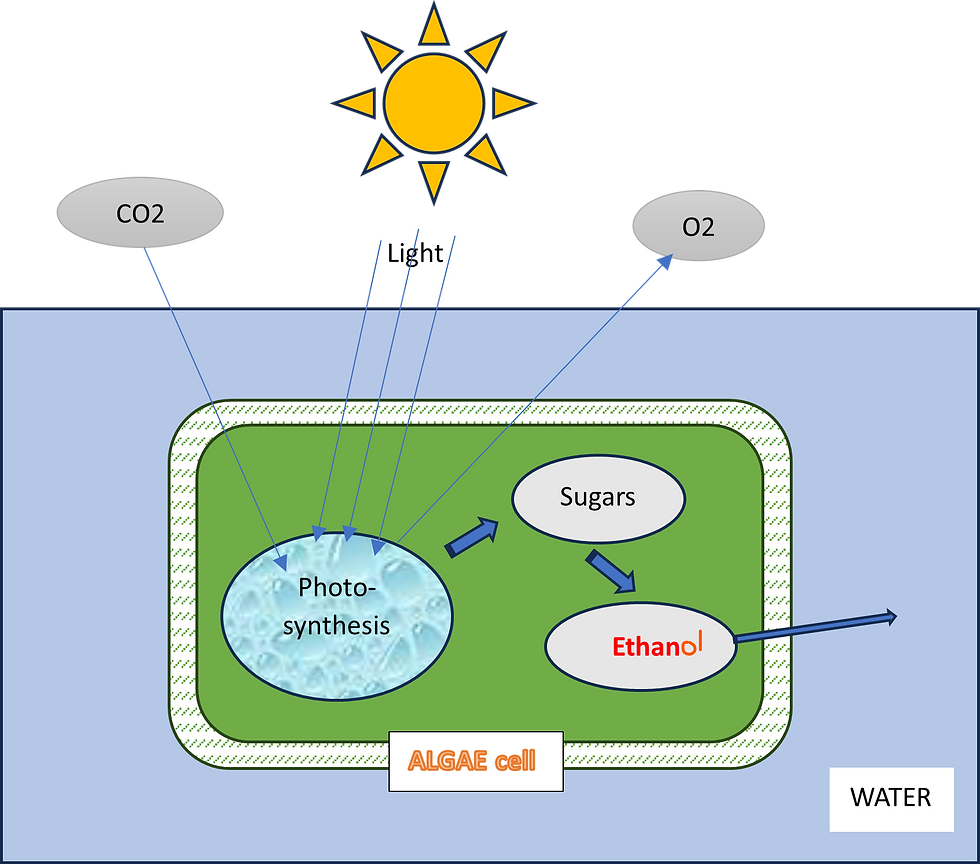
Upstream photosynthetic process
1. Bioburden is a major challenge. It is impossible to sterilize large-size field equipment. Need a breakthrough idea, like phosphorus depriving of bacteria method (if it works?) or an enzyme that restricts the growth of all species except our working strains.
2. Longevity of fermentation. The root cause problem is still to be recovered. A possible reason is the cell photo-inhibition or “Photon stress”. Concentrate effort on selective reduction of UV irradiation and keeping “mild” red-green) productive irradiation flux at maximum. Possibly staging critical experiments with various filters cutting different parts of the solar spectrum.
3. Productivity. Look for strains and conditions when maximal (as known in literature) growth rate can be achieved at first for non-GMO (Genetically Modified Organism) and then for GMO-ethanologenic strains. It looks like the “carbon switch” works well enough and Carbon efficiency during ethanologenesis is practically equal to the biomass growth rate. The idea is that fast biomass producers will generally be more ethanol-productive. Note. Estimation of productivity as % of PE/PAR (Photo Efficiency/Photosynthetically Active Radiation) is good for scientific research but for business-oriented engineers, a more realistic metric is ethanol growth in g/L/hr. Another important moment is the definition of productivity: plant ethanol production in gal/acre/year is not convenient to evaluate productivity reached in demo plant and so on.
4. Oxygen evolution. At the first stage of development, the level of dissolved O2 equivalent to pure O2 atmosphere should be accepted as inevitable. Need to exclude (avoid) air stripping during the production phase and especially nitrogen stripping: both methods, even if they have some effect, are economically impractical. One of the approaches will be considering shorter tubular reactors.
5. Oxygen scavenging. At some point need to check the scavenging option with SO2 (sulfite) using reaction [SO3=] + ½ O2 = [SO4=]. Small amounts of sulfite should be sufficient to reduce O2 levels and still not be toxic for cells. From in-house experiments, we know that [SO3=] conc > 10 mmol/L (600 ppm) kills cells quickly. But O2 solubility in water is only about 0.2-0.4 mmol/L and an equivalent amount of sulfite will be enough. The SO2 method is widely used to remove oxygen from boilers.
6. Oxygen should be the only gas present in the bio-reactor. No air stripping in PBR (Photo Bio Reactor) capsule/tubes and no CO2 gas-phase injection. It is technically impossible to equally distribute gas into multiple tubes without individual injections. Also gas displaces liquid in capsules and significantly increases PBR’s dark-volume. Concentrate efforts on the liquid phase process when CO2 is provided to the cells in the form of bicarbonate. This approach also directly leads to near-100% CO2 utilization that theoretically cannot be achieved in the gas-phase CO2 feed method.
7. Buffer for pH control. Stop using TRIS and switch efforts to serious investigation of bicarbonate-based media. At first, Nature invented a BIC buffer that provides optimal pH for algae in the seawater (pH=8.25). Secondly, it is known that cells can consume BIC with at least the same efficiency as dissolved CO2. Even good productivity results obtained with TRIS or similar organic buffer are not scalable: neither biologically nor technically and will lead to incorrect reactor design and separation technology. The buffer concept is closely related to the pH control technique.
8. Carbonic anhydrase seems (multiple papers) to play a critical role in CO2/BIC transformations, metabolism, etc, and deserves special R&D effort (study program).
9. PB Reactor design is a fundamental problem. Some literature surveys state that currently none of the existing PBR- Photo Bio Reactor models (solutions) can be considered economically viable. Any company (including JUT) that wants to succeed needs to come forward with a practical invention. A few criteria should be satisfied: efficiency, cost, longevity, deployment, reliability, etc.
10. Thermal management. Analysis by Uhde had shown that cooling costs are about 50% of all plant costs. Maybe they are not right but the present situation with PBR cooling leads to the conclusion that such plants can be sustained only in the coastal area where cooling is provided by the sea (lake) water taken from ocean depth. There is an associated challenge of corrosion that needs to be mitigated. Note. Experimental results with vertical reactors that indicate that such reactors need heating instead of cooling do not look convincing and probably need to be revised and/or repeated. It is even worse would they were found correct.
CO2 as a major feed component.
11. Flue gas requires purification from NOx and SOx and cannot be used directly as a feedstock, independently of its source. Joule experiments had shown that even moderately SO2/NO2 spiked CO2 gas kills cells quickly (one day in the fermenter). A study of the influence (toxicity) of other impurities like mercury, and HF that are normally present in coal-originated flue gas was not yet performed. There are 2 ways to deal with it: a) Buy pure liquid CO2 (CO2 could be not so expensive as thought but this approach defies the main idea of CO2 sequestration and “being green”) or b) Purify flue gas as part of solar ethanol plant design.
12. It is also desirable to develop a Flue Gas purification process that will include the production of near-pure CO2 free of inert ballast N2/O2. Operation on pure (>95% CO2) leads to multiple advantages:
a. No need for plant-level CO2 distribution piping network: CO2 can be delivered to each circulation unit in liquid form in pressurized tanks that will be enough to operate 10-acre CU for 5-10 days;
b. Ability to produce BIC locally by CO2 scrubbing with culture itself. It becomes impractical to use CO2 scrubbing when a substantial amount of inert gas (N2) presents in CO2 due to high losses of CO2 and water into the scrubber’s vent. Meanwhile, scrubbing pure CO2 by culture itself can be nearly 100% efficient.
13. The best arrangement would be to get pure liquid CO2 from a partnering Flue Gas Producer company (e.g. cement or coal plant). Public opinion on Global Warming and Green House Gas effect is developing in such a way that big CO2 emitters will start being penalized sooner or later. Thus, it would be good to reach such an agreement that Flue Gas Producer/Emitter would invest in its own CO2 recovery and purification plant, while Joule commits to acquiring significant volumes at a moderate price. (Both parties look “green” now). An alternative scenario when Joule builds its own CO2 plant is less attractive since Joule will be obliged to consume all the “raw” flue gas it gets from the Producer. Otherwise, Joule becomes responsible for the emission of all non-consumed CO2 (the difference between received and consumed).
CIP and SIP
14. CIP and SIP (Clean-in-Place and Sterilization-in-Place). Reliance on Hydrogen Peroxide (H2O2) as a principal disinfecting agent has already led to prohibitive costs. Using 1M peroxide for CIP and 2M for SIP results in the requirement of 12-15 MT of neat H2O2 per batch in 1-acre plant. If peroxide is acquired and transported to the plant as a 35% aq solution, it gives 50 MT per batch or 300 MT/yr (6 batches X 8 weeks). With ethanol productivity or 30 MT/yr/acre (1 gal=3kg), it means 10 kg of peroxide solution per 1 kg of product ethanol (it is not even funny!). Economics is prohibitive, not forgetting the safety measures required for transportation, storage, and security of such an amount of peroxide. There are 2 ways to deal with this: look for other sterilant or reduce peroxide consumption at least 100-fold. Eventually, it is possible considering that bacteria are killed not by H2O2 but by short-living radicals that are produced during the decomposition of H2O2 (like OH° and OH2° ). Thus, it is necessary to create a powerful flux of such radicals decomposing peroxide as fast as possible. People do it by using the so-called Fenton reaction when the peroxide decomposition reaction is accelerated by salts of iron (e.g., FeCl2). When uncontrolled Fenton reaction leads to a peroxide explosion but in our case it might be helpful (developmental work is required). Notes: a) Current Joule practice to add EDTA to peroxide solution produces quite the opposite effect and stabilized H2O2 (slows down its decomposition): EDTA chelates any traces of Fe. b) In all cases usage of Sodium Percarbonate as a source of H2O2 is much safer compared to aqueous peroxide.
Downstream recovery process:
15. Reactor design and recovery method selection are interdependent and cannot be developed by different departments (groups) without close coordination. It is desirable to assign one person with ultimate responsibilities for the plant design. His/her functions will be mainly coordinating efforts of biology (strain development), bioprocessing (reactor design and optimization), and engineering (plant hierarchical design, recovery, purification, recycling).
One of the examples of inter-department mutual dependency would be choosing of recovery concentration (working titer): Low ethanol titer in culture can be beneficial from a productivity point (Le Chatellier principle) but will lead to high recovery costs (stripping). Another example could be deciding on the addition of an anti-foaming agent to the culture: multiple effects on biology and downstream processing. Another is the selection of reactor temperature as much as biology permits: e.g. between 30 and 37oC.
16. Primary ethanol recovery from culture is a critical design decision that requires true ingenuity: invention(s) or a smart combination of known techniques. Recovery of ethanol from much-diluted solutions (0-2%) needs to be performed economically and continuously (Joule’s cornerstone ideology). Corn and cellulosic ethanol producers start recovery at 10-13% ethanol titer in fermented biomass and still require subsidies to match the manufacturing costs of synthetic ethanol. Of course, even matching the current recovery costs of corn ethanol will be a great victory for the “solar ethanol” idea. There are a few potential options to recover ethanol from culture: stripping, extraction, membrane selective permeation, solid phase adsorption, chemical… and the first question is whether the cells can survive the “treatment” and keep producing.
a. Less harmful seems to be air stripping (and it is the most studied process in JUT) but it is very expensive in both OPEX (energy for blowers) and CAPEX (cost of blowers and adsorbers). The high titer is highly desirable for air stripping. Anyway, stripping-adsorption is currently the only proven method that works. More effort can lead to process optimization and it is worth developing a comprehensive recovery model based on this technology and keeping it as a “base case” to compare with other methods. Investment in the stripping-related process is desirable.
b. Extraction from culture by the organic solvent is a highly unlikely option: first, a good solvent with reasonable selectivity to water-ethanol is yet to be found; second, the amount of solvent to extract ethanol would be comparable to the amount (volume) of water in PBR that implies significant investment into solvent recovery system at the CU level; third, most solvents are flammable and safety precautions dealing with them could have prohibitive costs.
c. Membrane separation is attractive (eventually not harmful to cells) but hits a fundamental problem: ethanol molecule is bigger than water and the physical size exclusion membrane will not work. The driving force should not be molecule size but some other phenomena. Other issues are membrane fouling and sterility.
d. Solid phase adsorption-based method includes the addition of solid material (dispersed) to the culture or passing culture through an adsorbing bed (like ion exchange) assuming this material has a strong affinity to ethanol. The first candidates will be special hydrophobic activated carbon or specially designed zeolite (of course should be cheap). Despite all foreseen difficulties this direction seems to be the most promising and worth of effort to investigate. Potentially SPA can deal with much-diluted solutions of ethanol and, thus, be applicable in case that low ethanol titer (e.g. <5 g/L) will be proven to be the most productive and not toxic to cells.
e. The probability of finding a chemical method to convert ethanol to something easily separated from culture is very low.
17. From “Beer” to “fuel grade” ethanol. It seems reasonable to think that primary recovery and capture of ethanol will result in an aqueous 10-15% solution (so-called “beer” grade) and then the process design of “secondary” recovery technology from such “beer” to fuel-grade (99.5%) can be delegated to the third parties with experience in this area. It might be true but a few moments are worth consideration:
a. In-house expertise in secondary recovery still needs to be preserved at least to be capable of evaluating the third parties' bids.
b. In-house experimentation in recovery cannot be abandoned. An experiment is the only way to address potential specifics of the JUT beer solution and make contractors aware of impurities, additives, etc.
Note: JUT's experience of ethanol sample preparation for German Automaker has revealed the presence of multiple and mostly unknown impurities in bioethanol. Some of the impurities tend to crystallize during distillation or color the product and cannot be ignored when designing or selecting recovery technology.
c. Total reliance on third parties and “lack of interest” to fuel grade recovery can exclude from consideration complex solutions that, for example, include simultaneous recovery from culture and getting “pure” ethanol.
d. Do not accept easily (naively) statements of contractors or engineering consultants that claim one or other technology to be an “industrial standard” – standards prone to change. For example, molecular sieves are claimed to be such standard for ethanol dehydration, but few papers show the economical advantages of extractive distillation with ethylene glycol or similar compounds (entrainers) that “break” azeotrope.
18. CAPEX vs OPEX. Technologies or design solutions should be compared and accepted or rejected on an economical basis and the best decision parameter would be the manufacturing cost of a gallon or kilogram of fuel-grade ethanol. Manufacturing cost is a function of OPEX and CAPEX and also depends on the production capacity of the designed facility and accepted capital depreciation time (e.g. 15 years). Separate minimization of OPEX or CAPEX leads to incorrect decisions in favor of one or another technology. A simple example would be a situation when energy requirements (low OPEX) can be reduced by implementing an energy integration system (high CAPEX). In special cases, technology can be rejected (not accepted) based on one part of the equation only: prohibitively high OPEX or CAPEX.
Recycling
19. Recycling. Sometimes there is insufficient clarification on the definition level of what recycling means (what is recycled?) and how it will be measured:
a. Water recycling. There is no question that water recycling should be maximized. In most areas with high insolation water resources are scarce and the cost of water would be high. A measure of recycling efficiency is not a percentage but absolute annual consumption (requirement) of fresh water by the solar plant in gallons or specific water consumption gallons per gallon of product (ethanol). This suggests that the Water treatment plant is an imminent part of the whole Solar plant. If this is not the case and the Solar plant sends wastewater for treatment to another Company, the cost of water treatment should be added to OPEX. Also in this case the ratio of waste water volume to the fresh water requirements could be calculated and used as a measure of water usage efficiency.
b. Media recycling. Here also 3 definitions can take place:
1) Recycling of nutrients (salts, buffer, cumate, antifoam, etc). In general cost of media can constitute a substantial fraction of manufacturing costs (as RawMat_cost = OPEX) and it is desirable to recycle at least part of these chemicals. For each chemical, a decision on its recycling should be taken on an individual basis (cost of raw material vs cost of recovery). Of course, some chemicals are partially consumed, and degraded and recovery cannot be 100%.
2) Media recycling from batch to batch when cells are separated and liquid is sent to the next batch to inoculate with replenishing of consumed or degraded nutrients. An important nuance here is a conceptual agreement on whether recycled media should be used entirely by the next batch in the same CU or can be sent to the common pool for processing and later distribution to any CU. The efficiency of such media recycling is limited by cell separation technology: for example, if a centrifuge is capable of concentrating cells from 3 g/L DCW to 30 g/L maximal media recycling will be 90%.
3) A special case of media recycling is recycling without post-batch ethanol separation. Now special measures should be undertaken to preserve ethanol from cannibalization by other microorganisms and also to design strains that will grow fast enough in ethanol-containing culture.
Note. This idea was pursued in JUT for about 18 months and quietly abandoned.
When water recycling and recovery/reuse of nutrients are logical and necessary [options a) and b1)], recycling of culture with or without ethanol poses significant biological and technical challenges:
a) potential accumulation of cell metabolism products in a culture that can be toxic to new cells.
b) Problems with keeping media sterile
c) Preservation of ethanol from degradation
d) Removal of carbon switch chemical (cumate aka p-hydroxyacetophenone) before inoculation
e) Complexity of nutrient replenishing and associated additional storage and chemical processing equipment.
Thus, a much more straightforward decision would be to rely on post-batch technology that will include immediate separation (recovery) of residual ethanol and deactivation of cells (killing), e.g. live steam ethanol stripping that will also kill cells. Then proceed with the recovery of nutrients and water purification.
20. Processing of biomass may represent a more or less challenging problem depending on applied regulations and the GMO status of Joule strains. If it may be possible to obtain regulatory permissions to treat solar plant biowaste as GRAS or “safe to environment” material, then after concentration and deactivation cells can be landfilled or drained somewhere. Otherwise, if classified as GMO, cells need to be almost completely dewatered (dried) and incinerated. Drying is a very energy-consuming and costly operation. Another “story” is DNA destruction requirements.
It is known that cells can be separated from culture by centrifuging or TFF (tangential flow filtration). Due to the very small difference in cell and media density centrifuging requires high G-force and significant centrifuging time. Significant improvement can be achieved by preliminary broth flocculation (with aluminum sulfate) but it complicates future media recycling. Also, centrifuging is not 100% efficient and some cells will inevitably get into centrate. TFF practically guarantees 99.99% cell separation but is expensive and requires periodic membrane cleaning from fouling.
21. Safety cannot be taken so easily and just rely on consultants that will do all the job. Consultants can give valuable advice but never accept any responsibility. A permanent safety engineer is required at the very beginning of the project (plant design) and needs to approve all URS, FRS, and so on. A good example is the usage of hydrogen peroxide which could be vetoed by the safety engineer in a JUT situation and would save a lot of effort. Emissions of Ethanol (HAP-Hazardous Air Pollutant and VOC-Volatile Organic Compound solvent) and potentially other components should be addressed at the plant design stage; a calculated and clear policy about emissions and their treatment (control) is required. It is not enough to say that” we will get a permit” or “this country/state does not require a permit” and we can operate as is. Practice shows that local authorities are very cooperative when a plant is going to be built in their jurisdiction but get more “environment protective” after hundreds of millions are already invested and the plant is ready to operate. It is very easy to vote and impose on the manufacturer-new emission regulations.
Modeling and simulation
22. Modeling and simulation. A few levels of models need to be developed for the solar plant:
a) Individual unit operations: PBR as plug-flow reactor, heat exchangers, and columns should be modeled using A+ with special packages for rigorous simulation when available. For example, Aspen PDR package for plate and frame heat exchangers, RATEFRAC for packed columns, etc. Development of homemade models of such a class is impractical and will require several man-years that exceed the resources of any start-up company. Betting on universal programming packages like MATLAB will lead to inevitable simplifications; especially difficult would be to develop physical properties packages applicable to the systems of interest present at solar plants: mixed solvents plus electrolytes. Other challenges include the necessity of documenting software and its testing. Media simulation requires using state-of-the-art electrolyte packages to predict solubility limits and eventual precipitation of mineral components as a function of concentration, temperature, and pH. This also includes modeling of bicarbonate buffers.
b) Using the Aspen Dynamics package for hydraulics simulation of complex piping constructions like fractal headers and pressure-driven systems like PBR sump reactor that works in conjunction with downstream adsorption system. This model should include a simulation of pressure relief devices and major valves. Specialized dynamic package ADSIM should be used to model adsorbers: carbon beds and molecular sieves.
c) Simulation of circulation unit (CU) as a batch operating system would be best performed in the Aspen Batch+ package, which provides convenient tools to model recipe-driven processes. Batch+ uses simplified models for unit operations but being once programmed (meaning to define a batch process as a recipe – sequence of operations) generates a plethora of valuable information: Material and energy balance, BOM, emissions, labor requirements, equipment cost, equipment allocation, Capex and Opex. As soon as Batch+ model one CU is built it can be “cloned” for a cluster of 8-12 CUs that share a seed reactor, CO2 purification unit, CIP tanks, waste collector tank, etc. After that cluster (or sub-plant) can be also modeled as a whole system for a time period including multiple batches (maybe a year). Results will provide information for the design of necessary shared facilities: cooling towers, boilers, CIP systems, seed reactors; their capacity, reservation, and bottlenecks. The same model could be used for what-if analysis: e.g. what happens if CU ends the batch prematurely.
d) Modeling of the whole plant also can be done in the Aspen Batch+ but will require further simplification of models of CU clusters. The result of such simulation will provide information for the design of the central plant and its shared facilities like recovery system, wastewater treatment, CO2 processing (if centralized at the plant level), product inventory, and required storage capacity.
e) Notes.
- Meaningful modeling of any type of PBR requires knowledge of reaction kinetics (in or case of photosynthetic growth of cells and further ethanologenesis). Such kinetic models could be obtained as a result of processing data from specially staged kinetic experiments or statistical analysis of experimental data obtained in other PBR experiments on condition that they cover a sufficient variety of process conditions. - Modeling at CU-level and higher (cluster, plant) makes sense only when all elements of process technology are known to the designer (what happens with each process stream) at least for one process option. All elements of such an option should be feasible and ultimately lead to the final product with specified characteristics. Meanwhile, the development of unit operations models and properties packages can be justified at the early stages of design: for example, cell separation can be modeled by centrifuging and TFF to compare this method on an economical basis.
f) 3D modeling of the whole plant is highly desirable.
Dmitry Vinograd
Aug 2014, amended Mar 2015,
Comments